Introduction to High Temperature Alloy Casting Processes
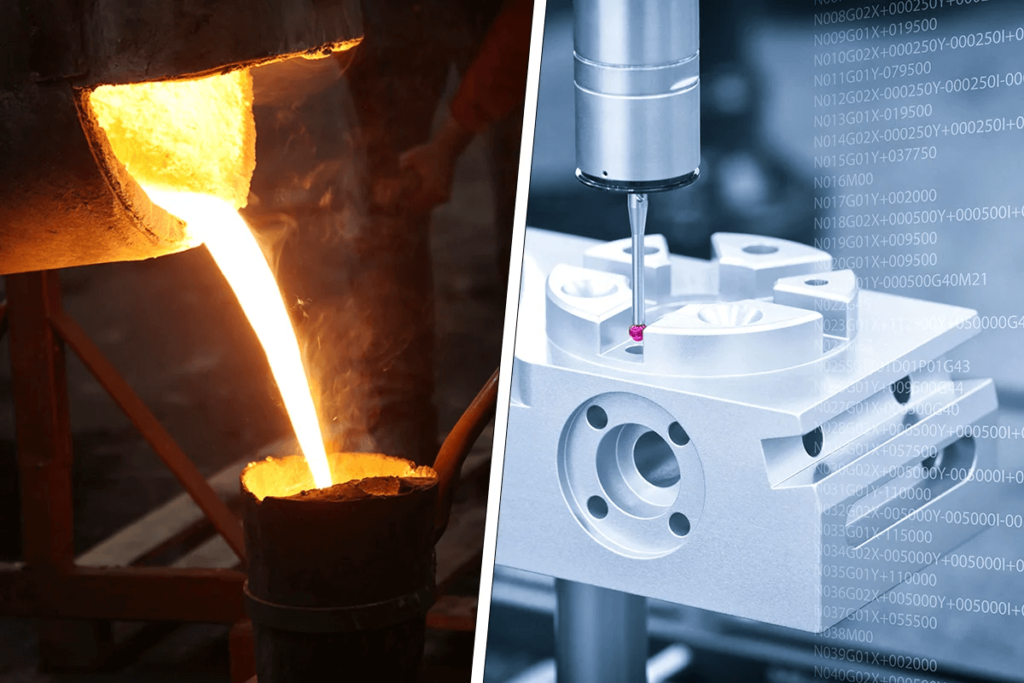
When it comes to manufacturing high-performance components, the high-temperature alloy casting process stands out as a critical technology. Why? Because industries like aerospace, automotive, and power generation demand materials that can withstand extreme heat, pressure, and wear. Think of jet engine turbine blades enduring fiery combustion cycles or industrial furnaces operating at temperatures that could melt standard metals. It’s no exaggeration to say that casting high-temperature alloys forms the backbone of modern engineering marvels.
But wait! Why do we even need high-temperature alloys in the first place? Their unique properties—such as high melting points, exceptional strength, and resistance to oxidation—make them indispensable in high-stress environments. Take a moment to imagine the consequences of using subpar materials. Cracking under stress, erosion from heat, and eventual failure—these scenarios are not just inconvenient; they’re catastrophic, both financially and operationally. That’s why understanding the nuances of these casting processes is not just a technical endeavor—it’s a necessity for businesses aiming for excellence.
Here’s the kicker: the high-temperature alloy casting process is not a one-size-fits-all approach. There are multiple techniques, each with its strengths and limitations, tailored for specific applications. From investment casting for intricate designs to sand casting for larger, less complex components, the options are varied but purpose-driven. If you’ve ever wondered why a particular turbine blade looks almost too perfect, chances are it was precision-cast using advanced methods.
What’s more, these casting processes are steeped in innovation. Technologies like additive manufacturing are revolutionizing the way molds are created, merging the precision of 3D printing with the robustness of traditional casting. As a result, manufacturers now achieve tighter tolerances, reducing waste while improving product reliability. It’s a fascinating synergy of age-old techniques and modern breakthroughs.
Now, here’s a pro tip: mastering the high-temperature alloy casting process isn’t just about understanding the methods. It’s also about appreciating the materials. High-temperature alloys, including nickel, cobalt, and titanium-based options, are the stars of this manufacturing show. Each alloy type offers distinct advantages, making the material selection process just as critical as the casting method.
So, let’s dive deeper. This guide will unpack everything you need to know about the high-temperature alloy casting process. We’ll explore its principles, delve into various casting methods, and discuss innovations shaping the future of this field. Whether you’re a technician, procurement manager, or curious reader, you’ll walk away with actionable insights. Ready to uncover the secrets of heat-defying manufacturing? Let’s begin!
Fundamental Principles of High Temperature Alloy Casting
The foundation of any successful high-temperature alloy casting process begins with a clear understanding of the materials involved and the principles that govern their behavior. High-temperature alloys are engineered to endure conditions where traditional materials fail, making them indispensable for advanced applications. But what exactly gives these alloys their exceptional properties? Let’s dig into the essentials.
First things first! High-temperature alloys owe their durability to their composition. Materials like nickel, cobalt, and titanium are at the core of these alloys. Nickel-based alloys, for instance, dominate industries such as aerospace because of their unparalleled resistance to oxidation and high-temperature creep. Similarly, cobalt-based alloys are celebrated for their wear resistance, making them ideal for cutting tools and medical implants. Titanium, on the other hand, offers a superb strength-to-weight ratio, which is why it’s favored in automotive and military applications.
But there’s more to it. The unique properties of these materials are further enhanced by the addition of elements such as chromium, molybdenum, and tungsten. These elements act as stabilizers, fortifying the alloy’s structure against thermal degradation and ensuring a longer service life. It’s this careful selection of components that allows high-temperature alloys to perform flawlessly in demanding environments, from jet engines to nuclear reactors.
Here’s a common misconception: It’s not just about the material. The casting process itself plays a pivotal role in realizing the full potential of these alloys. Casting is the art of turning molten metal into precise shapes, and it’s no small feat when dealing with materials that melt at extraordinarily high temperatures. Specialized furnaces, often lined with ceramics to resist heat, are required to maintain the purity of the molten metal. Even minor contaminants can compromise the alloy’s performance, so every step of the process is executed with meticulous precision.
Now, let’s talk about the physics at play. When molten metal is poured into a mold, it undergoes rapid cooling and solidification. This phase transformation is critical because it determines the alloy’s grain structure. A finer grain structure often translates to better mechanical properties, such as toughness and fatigue resistance. To achieve this, techniques like directional solidification and single-crystal growth are employed. These advanced methods ensure that the final product not only meets but exceeds the stringent demands of its application.
And here’s the kicker: Casting high-temperature alloys isn’t just about melting and pouring. The molds themselves are a marvel of engineering. Depending on the casting method, molds can be made of sand, ceramic, or even graphite. Each material offers specific advantages, such as thermal insulation or the ability to capture intricate details. For example, investment casting uses ceramic molds to achieve a near-perfect finish, eliminating the need for extensive machining.
Finally, let’s not overlook the role of process control. Every parameter, from temperature to pouring speed, is monitored and adjusted in real-time to ensure consistency. Sophisticated software and simulation tools have become integral to this process, allowing manufacturers to predict outcomes and minimize defects. The result? A casting process that’s as much about science as it is about craftsmanship.
By mastering these fundamental principles, industries are not only pushing the boundaries of what’s possible but also setting new standards for reliability and innovation. Whether it’s a rocket nozzle braving the vacuum of space or a gas turbine powering a city, the role of high-temperature alloy casting is undeniable. Want to explore the specific methods behind these processes? Let’s move forward!
Major Casting Methods for High-Temperature Alloys
Understanding the high-temperature alloy casting process requires an appreciation of the various methods used to shape these advanced materials into functional components. Each casting method is designed to cater to specific needs, offering distinct advantages depending on the complexity, size, and application of the part. Let’s explore the primary techniques that drive innovation in this field.
Let’s start with investment casting. Often referred to as lost-wax casting, this method is celebrated for its ability to produce intricate shapes with unmatched precision. The process begins with a wax pattern that replicates the desired component. This pattern is coated with ceramic material, creating a hard shell once dried. The wax is then melted away, leaving behind a hollow mold. Molten high-temperature alloy is poured into this mold, solidifying into the exact shape required. What’s the big deal? Investment casting eliminates the need for extensive machining, making it the go-to choice for complex parts like turbine blades or medical implants. Plus, the smooth surface finish reduces post-processing time, which is a major cost-saver for manufacturers.
Next up is sand casting, a method known for its versatility and cost-effectiveness. Sand molds are formed around a pattern, and once the molten metal is poured, the sand can be broken away to reveal the final component. While sand casting is less precise than investment casting, it’s ideal for producing large and bulky parts where minute details are less critical. Imagine engine blocks or industrial machine components—this is where sand casting shines. Moreover, the use of reusable sand makes this method more sustainable and budget-friendly for high-volume production runs.
But that’s not all—enter die casting. This high-pressure technique involves injecting molten metal into a steel mold. The metal cools and solidifies rapidly, allowing for the production of high-strength parts with tight tolerances. The automotive industry frequently relies on die casting for components like engine housings and transmission cases. Here’s a pro tip: die casting is best suited for high-volume production because the molds, while expensive to create, are extremely durable and efficient over time.
Let’s not forget centrifugal casting, which leverages rotational forces to shape metal into cylindrical parts. As the molten alloy is poured into a spinning mold, centrifugal force pushes it outward, creating a dense and uniform structure. This method is perfect for manufacturing pipes, bushings, and other hollow components where strength and durability are paramount. It’s a method that showcases how physics and engineering come together to solve real-world challenges.
Finally, continuous casting deserves a mention. Unlike other methods that focus on individual parts, continuous casting produces a constant stream of alloy in desired shapes like rods or slabs. This method is highly efficient for applications where material uniformity and scalability are critical. Steel mills and alloy suppliers often employ this process to provide raw materials for further manufacturing.
Each of these methods has its own set of strengths and trade-offs. The choice depends on factors like the complexity of the design, the volume of production, and the specific properties required from the alloy. By tailoring the casting process to the application, manufacturers can achieve unparalleled performance and reliability. Ready to compare these techniques side-by-side? Let’s dive deeper!
Comparing Casting Techniques
Selecting the right method in the high-temperature alloy casting process is akin to solving a complex puzzle. Each technique brings its own advantages, but how do you decide which is best for your application? The answer lies in understanding performance metrics and aligning them with project goals.
Here’s a simple breakdown. Investment casting takes the crown for precision and complexity. If you need a part with intricate geometries and minimal finishing, this method is a no-brainer. However, it’s worth noting that investment casting can be more expensive due to the meticulous steps involved. On the other hand, sand casting offers a cost-effective alternative for larger, less detailed parts. It’s the method of choice for projects where scale trumps fine detail.
But wait, there’s more! Die casting excels in high-volume production environments. The durability of its molds ensures consistent quality across thousands of units, making it perfect for industries like automotive and consumer electronics. If you’re working with cylindrical parts, centrifugal casting offers unparalleled uniformity and strength, thanks to its use of rotational forces. Finally, continuous casting is the epitome of efficiency, particularly for creating raw materials that require further shaping and processing.
Here’s what’s crucial: The material properties and application demands play a pivotal role in this decision-making process. For example, aerospace components require precision, strength, and heat resistance, making investment casting an ideal choice. In contrast, infrastructure projects might favor sand casting for its ability to produce large, durable components at scale.
Understanding these nuances not only ensures the success of your casting project but also optimizes cost and performance. The real magic happens when you align the method with your specific needs. Let’s move forward and explore how innovations are shaping these processes for the future!
Innovations in High-Temperature Alloy Casting
The high-temperature alloy casting process has come a long way, and innovations are continually redefining its boundaries. Today, advanced technologies are enabling manufacturers to push the limits of precision, efficiency, and sustainability.
Let’s talk about additive manufacturing. This game-changing technology integrates 3D printing into the casting workflow. How does it work? By printing molds and patterns with extreme accuracy, additive manufacturing eliminates many traditional limitations. This hybrid approach not only accelerates prototyping but also reduces material waste. For instance, aerospace companies are leveraging this technology to create lighter and stronger components with intricate internal structures.
Another breakthrough is the development of advanced coatings and materials. These innovations enhance the durability of molds and tools, allowing them to withstand the intense heat and pressure of high-temperature alloys. Coated molds last longer and provide better consistency, translating to higher-quality parts and reduced production costs.
But that’s not all. Simulation software is revolutionizing process control. With tools like computational fluid dynamics (CFD), manufacturers can model the flow of molten metal and predict potential defects before they occur. Imagine being able to test and optimize a casting process without wasting material or time. That’s the power of digital innovation.
These advancements are not just theoretical—they’re reshaping industries. From aerospace to energy, the integration of cutting-edge technologies is enabling manufacturers to create components that are lighter, stronger, and more efficient than ever before. Now, let’s explore the quality control measures that ensure these innovations deliver flawless results!
Quality Control in Casting Processes
The high-temperature alloy casting process is only as good as the quality control measures in place. Defects in casting can lead to catastrophic failures, particularly in critical applications like aerospace or power generation. This makes rigorous testing and inspection an integral part of the process.
First, nondestructive testing (NDT) takes the stage. Methods like X-ray and ultrasonic testing allow inspectors to peer inside a component without causing any damage. These techniques are perfect for identifying internal flaws like porosity or cracks. For surface-level issues, dye-penetrant tests provide a quick and effective solution.
Next comes destructive testing. While it may seem counterintuitive, breaking components in controlled settings reveals invaluable data about their mechanical properties. Tensile strength, fatigue resistance, and hardness tests ensure the material meets stringent specifications.
Here’s the kicker: Modern quality control doesn’t stop at testing. Advanced simulation tools now allow manufacturers to predict and prevent defects during the design phase. By analyzing factors like cooling rates and material flow, these tools minimize errors and optimize the process. It’s a proactive approach that saves time, money, and resources.
With these measures in place, the final product achieves the reliability and performance demanded by high-stakes industries. Want to see real-world examples of these techniques in action? Let’s move to the next section!
Case Studies
Aerospace and energy sectors are prime examples of how the high-temperature alloy casting process drives innovation. In aerospace, turbine blades for jet engines are often crafted using investment casting. These blades must endure extreme heat and centrifugal forces while maintaining aerodynamic precision. Similarly, in the energy sector, gas turbines rely on high-temperature alloys to operate efficiently and safely under immense thermal stress.
These case studies highlight the versatility and importance of advanced casting techniques. By tailoring processes to specific needs, manufacturers achieve exceptional results. Ready to wrap it all up? Let’s summarize!
Conclusion
Mastering the high-temperature alloy casting process is more than just understanding methods—it’s about integrating materials, innovation, and quality control into a cohesive strategy. By selecting the right casting method and leveraging modern technologies, manufacturers can create components that set new standards for performance and reliability. As industries evolve, staying ahead in casting technology is not just an advantage—it’s a necessity. Thank you for exploring this comprehensive guide. Looking for more insights? Check out our FAQ below!
FAQ
Q: What are high-temperature alloys?
High-temperature alloys are materials engineered to withstand extreme heat and stress, commonly used in aerospace, automotive, and energy applications.
Q: Which casting method is best for complex parts?
Investment casting is ideal for intricate designs due to its precision and smooth surface finish.
Q: How do manufacturers ensure quality in casting?
Quality is ensured through nondestructive and destructive testing, advanced simulation tools, and strict process controls.
Q: What industries benefit most from these processes?
Aerospace, automotive, power generation, and medical fields heavily rely on high-temperature alloy casting for critical components.